Big Bang Confirmed
The existence of the Big Bang has been confirmed through multiple independent mechanisms to have occurred about 13.78 billion years ago. We can even measure the left-over temperature from the Big Bang in empty space.
Two of the greatest discoveries made in cosmology during the twentieth century were that the universe is flat (space is not curved), and the cosmological constant (space is now expanding at an ever-accelerating rate). These are important because they both imply that the universe had a beginning – that it is not eternal.
Space and time were both created at the big bang event and there was no space or time before the universe began. These findings both have extraordinary theological implications that will be explored in future posts.
The twenty-first century would produce some astounding discoveries as well which would add further credence to the big bang model which will now be explored.
Helium Abundance
Helium is the second element in the periodic table whose nucleus is composed of two protons and two electrons (hydrogen is the first element with a nucleus composed of only one proton).
The big bang theory stipulates that most of the helium in the universe was made during the first few minutes of the creation event. When the universe was one millisecond old it was composed of protons, neutrons, and electrons with the only element in existence being hydrogen. When the universe was about four minutes old, it had cooled down sufficiently for nuclear fusion to occur allowing protons and neutrons to fuse forming heavier elements.
About one-fourth of hydrogen in the universe was converted into helium during about twenty seconds before the universe became too cool for nucleosynthesis to occur. After that narrow timeframe, all other elements we know and are made from were produced much later in the nuclear furnaces of a star (except for tiny fractions of lithium, beryllium, boron, and deuterium (an isotope of hydrogen with a nuclear made of one proton and a neutron).
The exact proportion of hydrogen and helium was determined by the density of hydrogen and helium during the universe’s first few minutes. According to the cosmic background radiation, the primordial helium abundance (before star formation) was 0.2668 ± 0.00013 of the original mass of hydrogen as published in a recent paper.
This predicted mass based on theory can be tested against observation of the helium abundance in gaseous nebulae. A nebula is a giant cloud of gas which we can detect because a nearby star lights it up. These gas clouds have not condensed into stars through gravitational attraction and have been essentially unchanged since the start of creation. Measuring the helium abundance in gas clouds that are not contaminated by gas from nearby stars allows scientists to determine the actual value for helium abundance in the very early universe.
This experiment has been carried out by two different groups of astronomers. One achieved a helium abundance value of 0.2446 ± 0.0029 while another team produced a value of 0.2449 ± 0.0040. The remarkable agreement between the value predicted for helium abundance by Big Bang theory and the value observed by two scientific teams helps to verify the big bang creation model.
Deuterium Abundance
Hydrogen has a single proton in its nucleus orbited by a single electron. Deuterium is an isotope of hydrogen with one proton and one neutron in its nucleus orbited by one electron. According to the big bang model, all of the lithium and deuterium that exists today was produced during the first four minutes of creation. That means that the lithium in your electric car was all produced 13.78 billion years ago in a tremendous creation event.
Stellar burning uses up tremendous amounts of lithium and deuterium. Scientists compared the amount of deuterium and lithium predicted by the big bang creation model with the deuterium concentration of certain types of quasars. A quasar or “quasi-stellar object” is thought to arise from the energy emitted as gas falls into supermassive black holes. The energy emitted by these black holes is tremendous, and their luminosity can be thousands of times that of the entire Milky Way galaxy.
Quasar activity was greatest approximately 10 billion years ago, with the most distant known quasar being nearly 13 billion light-years away. The hydrogen gas seen in these very distant objects is left-over from the creation event and has been incorporated into star formation. The concentration of deuterium in these very distant quasars of a special class (Lyman alpha systems) can be measured through analysis of their light spectrum.
The big bang model predicts a primordial deuterium abundance of 2.606 ± 0.053 x 10^-5 of the universe’s original hydrogen mass. The measured quasar deuterium abundance result was 2.457 ± 0.33 x 10^-5. The Planck Collaboration team noted the remarkable agreement between the predicted value and the actual value and wrote that it represented a “remarkable success for the standard theory of BBN [big bang nucleosynthesis].
Galaxies Spreading Apart
The big bang theory states space was more contracted toward the beginning of the universe; therefore, galaxies should have been much closer toward the beginning. This means that very distant galactic clusters should be more densely clumped than more recent once.
The most recent super-telescopes have allowed astronomers to make these measurements and have indeed demonstrated the galaxies from the distant past are so tightly packed together that their mutual gravitational attraction pulls them apart.
The examination of hundreds of galactic clusters from about 12 billion years ago demonstrates that galaxies were much more densely contracted than they are now. Furthermore, there is a smooth progression of the density of galactic clusters showing increasing separation as the universe gets older and older. This spreading apart of galaxies is a visual confirmation of the big bang theory.
Expansion Velocity and Big Bang Prediction
We have known the universe is expanding for about a century – it was one of the greatest discoveries in science because it implied the universe had a beginning – a start when the universe started to expand. Only in the last few years has it been possible to accurately determine the velocity of various galaxies from each other and from us.
It turns out there are four precision methods for measuring cosmic expansion. The average of the four methods with more than 600 measurements yields a rate of expansion of 68.65 ± 1.21 kilometers per second per megaparsec (where a parsec equals 3.26 million light-years).
Knowing the cosmic expansion rate allows the determination of the age of the universe; if you know how fast the universe is expanding it is possible to go backward in time to determine when the universe started to expand. Using this method of going back in time, the age of the universe is calculated as 13.78 ± 0.26 billion years.
The expansion rate of the universe is also consistent with the abundance of long-lived radiometric isotopes like uranium-238 and thorium-232, and with the measured ages of the oldest stars.
Big Bang Confirmed through Cosmic Cooling
The temperature of the one-second old universe was many millions of degrees; it then cooled over time as the universe expanded. Because the universe is a closed system (it is not in communication with anything else), its temperature would not have fallen over time. There are two commonly used means for the determination of the universe temperate at various ages.
- The fine structure of spectral lines from the interstellar gas within quasar spectra
- The so-called Sunyaev-Zeldovich anisotropy arising from the scattering of photons by energized electrons in galactic clusters
When the age of the universe is determined using these methods from multiple different studies using different techniques, the results fall directly on the predicted values. One investigational group noted,
independently of theoretical expectations, measurements of the temperature-redshift relation of the CMB black-body temperature provide a strong consistency check of the current Big Bang paradigm.
Cosmic Inflation
One of the most important concepts in the formation of the universe is the process called inflation. The universe begins at an extremely hot state where all the four primary forces of physics (electromagnetism, weak nuclear force, strong nuclear force, and gravity) all had the same strength. Then as the universe cools, these forces would gradually break apart.
- The first break would separate the unity of forces into two forces (gravity and the strong-electroweak force).
- This would then break into three forces (the strong nuclear force, the electroweak force, and gravity),
- and finally into the four forces currently present.
The important consideration is that at each of these breaks, the universe lost some symmetry and becomes more disordered.
Alan Guth made one of the greatest discoveries in science when in 1981 as an MIT professor he proposed the universe expanded very rapidly during the very early phase of the universe – a process called “inflation.” This inflation was so rapid that for a brief time, space (not matter within the space) expanded at a speed greater than light. This inflationary period solved many problems associated with the first hot big bang models. But a theory is only as good as it can be verified or proven incorrect.
An inflationary event would leave a signature in the cosmic background radiation particularly in something called the “scalar spectral index” or n_s. A universe with no inflation would produce an n_s of 1.0 or greater, while a universe with a simple inflation event would produce a scalar index of 0.95. A universe with a complex inflation event would produce a scalar spectral index of 0.96 to 0.97.
The scalar spectral index has been measured by the WMAP and Planck satellites as well as the South Pole Telescope.
- The Wilkinson Microwave Anisotropy Probe satellite measured the cosmic scalar spectral index to be 0.9608 ± 0.0073
- The Planck satellite map of the cosmic background radiation produced a measurement of the scalar spectral index of 0.9603 ± 0.0073
- The South Pole Telescope came up with a measure of the scalar spectral index of 0.9593 ± 0.0067
These measurements suggest that there is less than a 1 in 900,000,000 that the universe did not experience inflation during its early history. There is now no reasonable doubt that inflation occurred which lends further credence to the big bang creation event.
This is important because the big bang model strongly suggests a beginning for all things and for time ex nihilo or from nothing – just as Scripture says.
Star Populations and the Big Bang
The age and makeup of stars provide further information concerning the big bang model. The big bang creations model predicts a hierarchy in star formation. The earliest matter in the universe consisted of 76% hydrogen, 24 percent helium, and trace amounts of lithium. That means the earliest stars should approximate this element concentration.
The problem was that astronomers were not able to detect a star without other elements in its mixture. However, these earliest stars were not detectable by telescopes as they initially formed when the universe was only about 400 million years old – or when they were about 13.4 billion miles away from the earth. No telescope currently exists with the power to measure the spectra of such stars in order to determine their composition.
Populations of Stars. The first generation of stars should have no heavy elements as all heavy elements are created in past stars from previous generations. Stars go through their lifetime burning hydrogen into helium, and then later in their lives, they burn helium into larger elements. Stars that are larger than our sun typically explode at the end of their lifetime and seed the surrounding space with their material which can be used to produce the next generation of stars; these second-generation stars will have heavier elements that were produced by their first-generation parents. Most of the stars in existence are second-generation stars.
The sun is a third-generation star and so has elements heavier than helium and hydrogen in their makeup. Through a quirk in nomenclature, the oldest stars in the first generation are called Population 3 stars – or Pop 3. Our sun is a third-generation star or a Pop 1 star.
Unfortunately, even our largest telescopes have great difficulty in observing individual stars at their great distance from us – about 13.4 billion light-years. However, astronomers have discovered three Pop 3 survivors from which they can obtain the spectral analysis. One of these three stars is about 13.6 light-years away making it an ideal candidate for the determination of heavy elements. Spectral analysis of this star shows it has 18.2 million times less calcium than the sun, while the other two Pop 3 stars have 500,000 and 250,000 times less iron per unit mass than the sun.
These observations lend strong support to the concept of Pop 3 stars – they really do exist in the place and time predicted. It will be up to next-generation larger telescopes such as the James Webb Space Telescope and ground-based telescopes with mirror sizes exceeding 100 feet to make more exacting observations of the composition of stars at great distances.
Ages of the Oldest Population Two Stars
Most of the stars in the universe are Pop 2 stars as we noted above. These stars are produced from the exploded remnant gases expelled from larger Pop 1 stars which produced heavier elements while burning hydrogen and helium.
Pop 1 stars that were greater than 10 times the sun’s mass and produce heavier elements all the way up to the heaviest elements including uranium and thorium. Pop 2
stars made from the remnants of these Pop 1 stars will also contain uranium and thorium. These two elements are radioactive, meaning they decay at a precise rate over time.
Some radioactive elements decay in fractions of a second while others decay over billions of years. It turns out that uranium 238 has a half-life of 4.468 billion years while thorium 32 has a half-life of 14.05 billion years. A “half-life” is the time when half of the original radioactive substance will have been converted to other elements.
Astronomers began looking for a certain type of Pop 2 star; one that was very distant indicating it was formed in the distant past near the creation event and ones which had the least metallic content which should be the oldest. They have successfully measured the uranium level to the non-radioactive elements europium, osmium, and iridium and thereby determine the age of a Pop 2 uranium-238 containing star as 13.2 ± 1.5 billion years. Several other Pop 2 stars have also been characterized producing an estimate for the age of the universe to be about 13.7 billion years.
This is another confirmation of the Big Bang model and provides additional strength to the universe creation event.
White Dwarf Stars
The cooling of white dwarf stars gives astronomers another means for the determination of the age of the universe. White dwarfs are stars which have exhausted all their nuclear fuel and are in the process of cooling down. As the white dwarf stars age, they cool down in a predictable manner over a predictable timeframe. The oldest white dwarf stars are the coolest and since the age of the coolest white dwarf stars can be calculated it is possible to know their age.
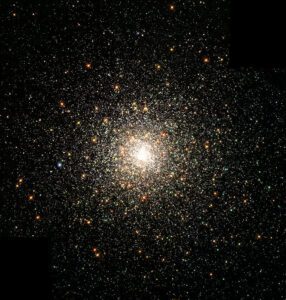
M4 Cluster
Using this technique, measurements of the nearest two globular clusters can be obtained. The nearest one, the M4 cluster, is 11.8 ± 0.6 billion years, while the second nearest globular cluster NGC 6397 has an age of 12.8 ± 0.6 billion years. When the formation time of these globular clusters relative to the big bang is added to their age, the age of the universe comes to about 14 billion years.
A deep dive into what these fascinating stars represent, how they are formed, and a deeper explanation as to how their age is determined can be viewed here for those who might be interested.
Primordial Magnetic Fields
Magnetic fields are typically measured in units of gauss or tesla, and there are 10,000 teslas in a gauss. One gauss is approximately the strength of the earth’s magnetic field.
Proponents of the big bang suggest that during the cosmic symmetry-breaking events (see above section) just after big bang, a small fraction of the energy released during these events might have been converted into what later became large scale magnetic fields exceeding about three light-years. There are currently no alternative explanations for these magnetic fields being present other than being associated with the big bang event.
Astronomers have found evidence for magnetic fields in all size scales except for the very largest. It turns out that the measurement of the relevant large-scale magnetic field is very difficult and will need to wait for future experiments. However, what can be said is that the early findings are supportive of the big bang model but certainly not yet conclusive.
Importance of These Observations
The hot Big Bang theory of the creation of the universe out of literally nothing has been one of the most controversial theories put forth during the last century. Initially, it was ridiculed and called “repugnant” or “crackpot.” For decades, scientists have tried to get around the theological implications of a beginning of the universe in a manner suggested by Scripture, most of which have been discarded when they could not be supported by observation. Most astronomers now reluctantly admit the cosmic origin likely occurred in a manner predicted by the Big Bang.
There are today only a handful of astronomers who are holding out for alternative explanations. Their resistance is based more upon philosophical and theological implications rather than upon what observations can test.